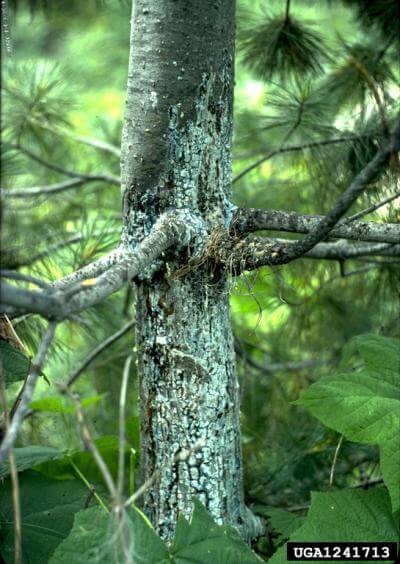
NOTE: this pest is not known to spread in or on firewood due to its complex life cycle requiring intermediate hosts. It is included in the Gallery of Pests for general information purposes only.
White pine blister rust Cronartium ribicola
White pine blister rust (WPBR) is a damaging disease of five-needle pines (genus Pinus section Quinquefoliae) caused by the rust fungus Cronartium ribicola. Epidemics of this introduced pathogen have produced severe economic and ecological losses to North American white pines. WPBR has caused drastic declines in white pines across the West. Currently attention focuses on high-elevation pines, especially whitebark pine, which is suffering extensive mortality from a combination of drought, attack by mountain pine beetle (MPB), and the introduced disease, WPBR. [Barrett, 2021]
For comprehensive reviews and synthesis of the history, ecology, and management of white pines threatened by white pine blister rust see the August 2010 issue of Forest Pathology (Vol. 40:3-4) and the 2022 conference, “Research and Management of High Elevation Five-Needle Pines in Western North America”. Access to the conference’s proceedings as available at Research and Management of High-Elevation Five-Needle Pines in Western North America: Proceedings of the Second High-Five Conference.
The Special Issue of Forest Ecology and Management (521; 2022) is available free of charge until mid-January 2023 at
https://www.sciencedirect.com/journal/forest-ecology-and-management/special-issue/10JN0Z5W6GX
All North American species of white pine are susceptible to white pine blister rust. In eastern North America, the pine host is eastern white pine (P. strobus). In the West, seven species of white pine are infected in the wild; these include: western white pine (P. monticola), sugar pine (P. lambertiana), whitebark pine (P. albicaulis), foxtail pine (P. balfouriana), limber pine (P. flexilis), southwestern white pine (P. reflexa), and Rocky Mountain bristlecone pine (Pinus aristata). Infestations have not been reported for Great Basin bristlecone pine (P. longaeva) and the Mexican white pine species. Western white pine and sugar pine usually occur in moist, mid-elevation forests (Schwandt et al. 2010). Southwestern white pine is most prominent on “sky island” peaks of the American southwest. Western white pine once dominated the canopy in Idaho and Montana; logging removed the valuable sawtimber trees and disease severely reduced reproduction (Schwandt et al. 2010). Sugar pine is the largest pine in North America. Western white and sugar pines could reach diameters of 30 inches dbh or greater and heights of 200 feet (Goheen and Goheen, 2014). The other western white pines occur in dry, timberline communities, where they have persisted for thousands of years under harsh conditions (Hutton, 2015). Many of the individual trees are long-lived – thousands of years in the case of the bristlecone pines. They play important roles in high altitude, unique ecosystems (Dawe, 2020; Hutton, 2015) such that:
- They retained ground water, slowed the rate of snow melt, and maintained stream flow characteristics and water quality;
- They curtailed soil erosion and maintained slope stability; and
- They provided high-value food and shelter to wildlife, in particular bears and nutcrackers.
USFS scientists and managers developed a conservation priority-setting framework for forest tree species, like white pines, that are at risk from pest, pathogens and other threats. The Project CAPTURE (Conservation Assessment and Prioritization of Forest Trees Under Risk of Extirpation) uses FIA data and expert opinion to group tree species under threat by non-native pests into vulnerability classes and specify appropriate management and conservation strategies. The scientists prioritized 419 tree species native to the North American continent. The analysis identified 15 taxonomic groups requiring the most immediate conservation intervention because of the tree species’ exposure to an extrinsic threat, their sensitivity to the threat, and their ability to adapt to it. Each of these 15 most vulnerable species, and several additional species, should be the focus of both a comprehensive gene conservation program and a genetic resistance screening and development effort.
The project CAPTURE process ranks sugar pine second in needing conservation and resistance breeding because the species is relatively rare, thereby more sensitive than some other pine species. Project CAPTURE places whitebark pine as having a “moderately high” mean pest Severity score but among the lowest Sensitivity and Low Adaptive Capacity scores; whitebark pine is not ranked among the 15 species most deserving conservation effort, perhaps because there is already a major conservation effort for this species (see species-specific profile below). In contrast, the U.S. Fish and Wildlife Service (USFWS) has proposed listing whitebark pine as a threatened species under the Endangered Species Act. An analysis of tree species’ conservation status by conservation organizations (Carrero et al. 2022) concluded that the species is vulnerable or endangered.
White pine bluster rust was introduced from Europe to North America in shipments of infected pine seedlings during the decades around 1900. Initially, the pathogen spread rapidly in the humid-temperate climates of the maritime and Great Lakes regions. Spread and intensification in the dry, extreme climates of continental regions and alpine environments has been slow, but by 2020, impacts were significant. White pines in Mexico and much of the southwestern U.S. have escaped infection, even though aecial and telial hosts are present, and other stem rust fungi tolerate the climate of these regions.
WPBR is most severe in smaller trees, that is the seedlings and saplings that are key to regeneration.
White pine blister rust requires a living host and alternates between two host types named for distinctive structures that produce the infective spores. White pines are aecial (cup-like) hosts; Ribes (currants and gooseberries), Pedicularis (lousewort), and Castilleja (paintbrushes) are telial (hair-like) hosts. Host plants are infected through needles or leaves by aerially dispersed spores. Spores produced on an aecial host infect only a telial host. One type of spore from a telial host intensifies the pathogen on other telial hosts; a different spore type spreads the pathogen to white pines. Parasitism of pine inner bark produces a necrotic canker than can eventually kill the host, while the disease only defoliates telial hosts.
Control of white pine blister rust uses silviculture and genetics to reduce infection and damage (King et al. 2010; Ostry et al. 2010; Zeglen et al. 2010). Early controls employed quarantine and eradication of Ribes; later, chemical and mechanical sanitary methods were developed. Current practices rely on vegetation management (site selection and preparation) and genetic selection to improve resistance. Numerous, site-specific factors affect the likelihood of infection and severity of infestation. Frequent episodes of cool, wet weather during the late summer favor inoculum production and dispersal. Proximity to telial hosts with abundant inoculum and abundant tree foliage close to the ground increase pine infection. Inherited traits affecting tolerance and resistance to infection vary by host species and population (these are described below). Interactions between environmental and genetic factors are also important (Richardson et al. 2010).
Western five-needle pines are also under attack by a native insect, the mountain pine beetle (MPB; Dendroctonus ponderosae). Beetle populations periodically erupt into epidemics that can kill nearly all mature hosts. Many pine species are hosts, including nearly all the five-needle white pines. Except in Arizona, California, and New Mexico, bark beetles are having a larger impact on forest CO2 emissions than is fire (Barrett, 2021).
Infection by parasitic mistletoe plants is a major cause of mortality particularly of pines in the Rocky Mountains (limber, southwest, and Rocky Mountain bristlecone pines). Mistletoe infection weakens trees and predisposes them to other threats such as MPB and drought (Schoettle, 2022; Hupp and Stucki, 2022).
Climate change is expected to create unprecedented climates across approximately half the West by the end of this century. These stresses could trigger changes in vegetation types and extent, net primary productivity, wildfire frequency, and expansion of the range of tree-damaging pests. The subalpine forest habitats of most of the western five-needle pines are expected to contract (Barrett, 2021) However, several sources conclude fire and climate change per se will have less impact than WPBR and MPB [FWS].
The USDA Forest Service (USFS) and cooperators (including in Canada) have put forth considerable effort to identify trees resistant to WPBR and, where necessary, breed trees with greater resistance. Initial efforts focused on western white and sugar pines, which have significant timber value. In recent decades programs have expanded to address the species that grow at high elevations. However, there are significant biological, logistic, and resource constraints. Sniezko and Liu (2022) conclude that breeding will not be attempted for most of these species. (See individual species descriptions below.)
Summary of status of host species:
Eastern white pine (Pinus strobus)
Eastern white pine is native to a broad range from Newfoundland, Canada west to central and western Ontario and extreme southeastern Manitoba; south to southeastern Minnesota and northeastern Iowa; east to northern Illinois, Ohio, Pennsylvania, and New Jersey; and south mostly in the Appalachian Mountains to northern Georgia and northwestern South Carolina. It is also found in western Kentucky, western Tennessee, and Delaware. A variety grows in the mountains of southern Mexico and Guatemala (Wendel and Smith).
Before introduction of WPBR, eastern white pine was a major timber species in eastern Canada, New England, and the Great Lakes (Geils et al., 2010). While (Ostry et al. 2010) reported that WPBR is now a minor concern in growing EWP for ornamental or wildlife use, Wendel and Smith reported the contrary: that it is highly virulent throughout the tree’s range. Trees are susceptible from the seedling stage through maturity. Blister rust can cause high losses both in regeneration and in immature timber stands (Wendel and Smith).
While early attempts to breed trees resistant to WPBR were disappointing, in recent decades scientists have developed a new understanding of potential genetic resistance, so they have created seed orchards and begun long-term field tests to evaluate resistance (Sniezko & Liu, 2022).
Western white pine (Pinus monticola)
The range of Western white pine is from sea level in British Columbia, Washington, and Oregon into Idaho and Montana; and subalpine forests of northern California (Tomback and Achuff, 2010). White pine blister rust poses an existential threat to this species (Tomback et al. 2022). Due to the combined impacts of WPBR and overharvest, more than 90% of the historical white pine range is now dominated by fir, larch and western hemlock (Schwandt et al., 2010).
By the beginning of the 21st Century, across Washington and Oregon 32% of living pines were infected by WPBR, 10% infested by MPB. In southwest Oregon, half of the species’ total basal areas was comprised of dead trees (Goheen and Goheen, 2014). In Yosemite and Kings Canyon/Sequoia National parks in California’s Sierra Nevada Mountains, WPBR had moved to higher elevations – although decline of western white pine was not as severe as that of sugar pine (Nesmith et al. 2022).
The USFS began efforts to breed western white pine resistant to WPBR in the 1960s. Initial success relied on single-gene- (MGR) based resistance; however, WPBR evolved to render that ineffective in at least part of the range. DGRC is now working with QR. The USFS Dorena Genetic Resource Center (DGRC) has evaluated resistance in more than 4,000 parent trees of western white pine. Resistant seed from the first orchards is now being used in reforestation (Sniezko pers. comm.).
Sugar pine (Pinus lambertiana)
Sugar pine is the largest pine in North America, with considerable timber, aesthetic and ecological value (Tomback and Achuff, 2010). Its range reaches from southern Oregon to northern Baja California. WPBR infestation is restricted to the Sierra Nevada and farther north.
As of the first decades of 21st Century, in Oregon and Washington, combined, 32% of living western and sugar pines were infected with WPBR, 10% with MPB. In southwest Oregon, 13% of standing sugar pine biomass was dead (Goheen and Goheen, 2014).
In California’s Sierra Nevada Mountains, there have been dramatic declines of sugar pines since the 1990s due to the combined effects of MPB, drought/fire, and WPBR. The number of trees per hectare was halved, driven by mortality of 52% of the smaller, young trees dead. Of the sugar pines infected by WPBR in the 1990s, 74% had died by 2013. (Nesmith et al. 2022).
The USFS began efforts to breed sugar pines resistant to WPBR in the 1960s. Initial success relied on single-gene- (MGR) based resistance; however, WPBR evolved to render that ineffective. The DGRC is now working with multiple-gene or quantitative resistance (QR). The DGRC has evaluated resistance in more than 4,000 parent trees of sugar pine. Resistant seed from the first orchards is now being used in reforestation (Sniezko pers. comm.).
Whitebark pine (Pinus albicaulis)
Whitebark pine has the largest range of any of the five-needle pines in North America. [Nesmith et al. 2022]. It includes high elevation slopes in the Rocky Mountains, Coast Range, and Sierra Nevada-Cascades (Tomback and Achuff, 2010). In the northern Rockies whitebark pine’s range reaches from southern Greater Yellowstone (~42o North) to about 55 o North in Canada (Tomback et al. 2022). This range equals more than 30 million hectares (~more than 81,000,000 acres) (Tomback et al. 2022) citing COSEWIC (2010); USFWS proposal]. Estimates of the proportion of this total in the two countries varies considerably; the U.S. range is estimated at 15 million ha by COSEWIC (2010) but nearly 23 million ha by USFWS. Earlier estimates (Keane et al. 2012; Goeking and Izlar, 2018) had given much smaller estimates – of less than 6 million ha.
Before arrival of WPBR, whitebark pine had persisted for thousands of years under harsh conditions (Hutton 2015). Whitebark pines provide critical food for many wildlife species at high elevations —notably bears and nutcrackers; the latter play vital roles in the tree’s reproduction by distributing its seeds.
Whitebark pine is under the greatest pressure of all the high-elevation five-needle pines. The disease was first reported on whitebark pine in 1926 (Schoettle et al. 2022). By 2020, mortality of whitebark pine exceeded growth (Goeking and Izlar, 2018) WPBR currently infests ~34% of the tree’s range. By 2020 51% of all standing whitebark pine trees were dead; half of this mortality had occurred within the last two decades. This widespread mortality occurred in just one and one-half tree generations after the introduction of WPBR [USFWS]. Once the pathogen is present, there is no means of eradicating it. At present, damage is greater in the northern portions of the range. However, the USFWS expects the rust to continue spreading; within 60 years (1 generation of the tree), it is expected to spread to 52% – 88% of the range, depending on the spread scenarios. The intensity of infestation described here might be an underestimate; research in the Greater Yellowstone Ecosystem determined that more than 30% of trees had been infected in recent decades, not the ~20% as formerly believed (Shanahan and Irvine, 2022).
Viability of whitebark pine is further complicated by its vulnerability to attack by the mountain pine beetle (MPB). Currently ~17% of the range is affected; in the northern U.S., up to 25%. The USFWS expects the MPB to spread within 60 years to as much as 40% of whitebark’s range [USFWS]. While WPBR causes damage most rapidly on smaller trees, the MPB attacks mature trees; at epidemic levels, MPB can kill 80-95% of its hosts. These large trees are the seed producers, so MPB attack threatens reproduction. Compounds in that greater impact at northern parts of range where at least 25% of range affected (same area as most affected by WPBR) [FWS]. Interactions between the two pests also poses its own threat. In the Greater Yellowstone Ecosystem of the Northern Rockies and into Canada, ecologists worry that the shift toward smaller trees resulting from MPB-caused mortality of larger trees might increase the impact of WPBR. (Shanahan and Irvine, 2022).
In the Pacific Northwest, the combined impact of WPBR and MPB is expected to cause death of nearly half of the whitebark pines at Crater Lake and Mt. Rainier National parks; 40% at Cascade National Park (Jules et al. 2022).
It is particularly important to protect whitebark pine populations that are genetically distinct. These include the populations on the Olympic Peninsula (Novoa et al. 2022) and in the Sierra Nevada (Jules et al. 2022). Furthermore, stresses on the Sierra Nevada populations are somewhat different. Levels of WPBR are relatively low, fires occur less frequently (Jules et al. 2022). As a result, these whitebarks are currently healthier than trees in other parts of the range. But WPBR is spreading (Nesmith et al. 2022; Meyer et al. 2022) and is expected to increase at high elevations (Nesmith et al. 2022).
The effects of climate change is unclear. Most expect it to further threaten whitebark pine because the species is adapted to cool habitats and cannot migrate to higher elevations since it already grows at the top of ridges and mountains. In addition, it is uncertain whether whitebark pines can migrate farther North. Issues include the virulence of WPBR there and questions as to whether the nutcracker will migrate to those locations. Under these circumstances the USFWS expect climate change to significantly decrease whitebark pines’ persistence rangewide within 100 years (or less than two generations of the tree). In the Greater Yellowstone Ecosystem, some expect the species’ range to contract from 90 – 70% of the region to less than 18% due to changes in available water and rising temperatures (Hansen et al. 2022).
However, research in the Sierra Nevada found that drought led to better growth of whitebark pine at high elevations; apparently the drought and warmer temperatures alleviated temperature constraints on growth in very cold regions. Dryness also hindered spread of WPBR. However, at low elevations, in more water-limited areas, growth declined and there was significant mortality. Interactions with the other principal mortality agent, MPB, are highly complex/site-specific (Dudney et al. 2022).
Furthermore, USFWS has concluded that high-severity fire – even exacerbated by climate change – is not likely to impact severely whitebark resiliency, redundancy, or representation absent other factors.
For much of the 20th Century there was little effort to manage whitebark pine because it has no commercial value and grows in difficult-to-access areas (King et al., 2010). Increasing recognition of the species’ ecological value in recent decades has prompted considerable effort. U.S. federal agencies in the northern states have been managing whitebark pine for decades, employing prescribed fire, thinning, planting, and long-term monitoring (Guiberson and Byron, 2022; Voight and Murphy). The USFS began exploring resistance levels in whitebark pine populations in 2002; the Dorena center now collaborates with many stakeholders throughout the range. Crater Lake National Park began a conservation program in 2003 [Hooke]. American Forests engaged at a serious level beginning in 2012 [AF website] Stakeholders in the Crown of the Continent Ecosystem began working together in 2016. There is some hope for exploiting new funding sources – including the Infrastructure Investment and Jobs Act, America the Beautiful, 30×30, and Trillion Trees programs. American Forests is leading efforts to obtain funding from these and other sources [Sniezko 2022 webinar].
Whitebark pine has a sufficiently high level of resistance in some seed zones that seed collected from parent trees in the wild can be used without further enhancement for restoration. However, collecting whitebark seed crops is difficult, expensive, and chancy – due to sporadic seed production and threats from fire and MPB threats to mature trees – so programs are also establishing grafted seed orchards. (Sniezko and Liu 2022; refer to the chapter for a discussion of details, and what is required to sustain a program]. See also
https://www.fs.usda.gov/detail/r6/landmanagement/resourcemanagement/?cid=fsbdev2_027074)
The seeds of whitebark are dispersed to newly disturbed, open areas by Clark’s nutcracker (Nucifraga columbiana). Furthermore, whitebark cones open to release seeds only after fire. Therefore, many programs are trying to use prescribed fire to promote regeneration. However, both nutcracker seed caching behavior and seedling establishment are complex so the role fire plays depends on site characteristics, like openness and the presence of other tree species. For example, in Alberta, fire appeared to boost regeneration of the dominant tree species – rather than whitebark pine (Dawe et al. 2020).
USFWS listing
Recognition of the impacts of WPBR led to petitions to USFWS to list as endangered as early as 1991; the USFWS rejected this petition as not containing sufficient information to justify listing. Additional petitions resulted in the species becoming an official “candidate” for listing in 2011. The USFWS proposed listing the species as threatened in December 2020 [USFWS]. The final decision was supposed to be released within one year, but was not.
The USFWS evaluated five factors: (A) The present or threatened destruction, modification, or curtailment of its habitat or range; (B) overutilization for commercial, recreational, scientific, or educational purposes; (C) disease or predation; (D) the inadequacy of existing regulatory mechanisms; or (E) other natural or manmade factors affecting its continued existence.
In deciding to propose the species for listing as “threatened with extinction in the foreseeable future”, the USFWS defined the tree species’ generation as lasting ~60 years. USFWS defined “viability” as the ability of the species to persist over the long term (i.e., to avoid extinction). FWS did not propose a listing as “endangered” because the species is still widespread, and individual trees persist for decades [USFWS].
The proposal concludes that the primary stressor is white pine blister rust (Factor C). This threat is exacerbated by the mountain pine beetle (Factor C), altered fire regimes (Factor E), and climate change effects (Factor E). The Service notes strong synergies among the four key stressors are likely to exacerbate their combined effects [USFWS].
If the USFWS lists the species as threatened, it expects to develop a Recovery Plan. Emphasis will be on supportive silvicultural practices, e.g., removal of competing species, cone collection, planting, fire management, monitoring – probably with a specific rule under Section 4(d). Since 88% of the U.S. range is on federal lands, (Tomback et al. 2022) the USFS, National Park Service, and Bureau of Land Management, are obligated by Section 7 of the Endangered Species Act to carry out programs to conserve listed species.
In Canada, whitebark pine was listed by the Province of Alberta as endangered in 2008; however, this listing provided no regulatory protection. The Canadian federal government listed the species as endangered under the Species at Risk Act in 2012. The listing was justified by the threat from WPBR, MPB, changes in fire regimes, and climate change. The federal recovery plan was released in draft in 2015, but it had not approved as of summer 2021. In the absence of a recovery plan there is no protection for whitebark habitat; only individual trees on federal lands – nearly the entire range in Canada – are protected (Krakowski et al. 2022).
Bolstering rust resistance is recognized as key to recovery. Rust resistance screening is under way in various projects in both controlled and field setting. Collaborations with the White Pine Ecosystem Foundation (WPEF), USFS’ DGRC, Alberta and Canadian Forest services (genomics) (Krakowski et al. 2022).
Limber pine (Pinus flexilis)
Limber pine is distributed from the Canadian Rockies in Alberta and British Columbia to the southern Rockies in New Mexico and desert ranges in California. It grows in a wide range of ecosystems within these ranges – both upper elevation treelines and lower treelines in grassy, open forests; on exposed rocky slopes; and in dense, mixed-conifer stands. Before arrival of WPBR, limber pine had persisted for thousands of years under harsh conditions (Hutton 2015). Like other high-elevation five-needle pines, limber pine provides important ecosystem services, including critical food for many wildlife species at high elevations —notably bears and nutcrackers; the latter play vital roles in the tree’s reproduction by distributing its seeds (Tomback and Achuff, 2010).
WPBR was first reported on limber pine in 1945 (Schoettle et al. 2022). Limber pine faces an existential threat from this pathogen (Tomback et al. 2022) Trees of all ages are killed. Rangewide, mortality exceeds growth esp. of trees larger than seven inches dbh (Goeking and Izlar, 2018). The proportion of limber pines that are dead increased from 8% in 1999 to 34% in 2009 to 42% in 2019. Conservationists note that this trajectory mirrors that of whitebark pine a decade or so ago. (Schoettle et al. 2022).
The disease is common in northern parts of the range, generally absent in the south (Schwandt et al., 2010). Nearly 90% of the limber pine range in Alberta is affected (Dawe et al. 2020) WPBR distribution uneven; for example, at low elevations in southern Idaho (at Craters of the Moon National Park) there is very little WPBR (Stucki, 2022).
Other threats
Limber pine is a preferred host of MPB. Barrett and Robertson (2021) expect limber pine to be one of several species to suffer substantial levels of tree mortality in the near future due to MPB mortality (44% of total basal area). Another threat is dwarf mistletoe [Schoettle oral]
Conservation Efforts
Since, like whitebark pine, limber pine seeds of limber pines are dispersed to newly disturbed, open areas by Clark’s nutcracker (Nucifraga columbiana), restoration efforts often try to use prescribed fire to promote regeneration. However, both nutcracker seed caching behavior and seedling establishment are complex so the role of fire depends on site characteristics, like openness and the presence of other tree species. For example, in the Colorado Front Range, limber pine can be replaced by subalpine fir when fire-free intervals are long. On the other hand, in Alberta, fire appeared to boost regeneration of the dominant tree species – rather than limber pine (Dawe et al. 2020). Dawe et al. recommend protecting existing stands of limber pine through fire mitigation efforts, e.g., thinning and other fuel treatments, and supplementary planting of seedlings.
The DGRC began testing limber pine trees for possible resistance to WPBR in 2003. The program has since expanded to include hundreds of seedling families from populations in the U.S. & Canada. Most of the parent trees tested from U.S. sources are from areas with little WPBR, while most of the Canadian parent trees are from areas with very high levels of blister rust. Restoration plantings are using WPBR resistant seed collected from habitat [Sneizko].
In Canada, a multiagency recovery team was formed in 2003. The species was listed as endangered by Alberta in 2008 but the listing conveys no legal protection for listed plant species. Alberta did adopt adopted a recovery plan in 2013, which is now being consolidated with that for whitebark pine. Listing at the national level was recommended in 2014, but it has not been done yet (Krakowski et al. 2022).
Canadian scientists are also screening the species for resistance; they have also established seed orchards in partnership w/ WPEF, British Columbia and Alberta, and Parks Canada.
Sniezko and Lui (2022) expect that no substantial breeding work to be carried out for limber pine in the near future. Restoration efforts will have to take into account current levels of resistance.
A complication is that there probably will not be enough resistant seed or other resources to restore all the limber pine populations lost to WPBR. The solution appears to be strategic ‘nucleation plantings’ Sniezko and Lui (2022).
Southwestern white pine (Pinus reflexa)
Southwestern white pine is closely related to limber pine and sometimes hybridizes with it (Schoettle et al 2022). It range reaches from southern Colorado to Mexico. Southwestern white pine is an important component of the mixed-conifer forests upslope from pure ponderosa pine (Pinus ponderosa) but in the U.S. its distribution is fragmented among the “sky islands” of mountain peaks in the Southwest. This distribution makes the species highly vulnerable to fire, since entire populations can be killed by a fire (Schoettle et al. 2022).
WPBR was apparently first detected on southwestern white pine in 1990 (Schoettle et al. 2022) While the epidemic has intensified in the cool, wet Sacramento Mountains of southern New Mexico and is spreading, many populations are still healthy (Schoettle et al. 2022). Southwestern white pine has higher levels of resistance than sugar and limber pine [Sniezko] Southwestern white pine is experiencing growing mortality by fire [Goeking, and Windmuller-Campione 2022].
The USFS DGRC began testing southwestern white pine for levels of resistance in 2001; the program expanded in 2014. Seedlings from more than 400 parent trees, include nearly the entire range of the species, in both the U.S. and Mexico.
Sniezko and Lui (2022) expect that no substantial breeding work to be carried out for southwestern white pine in the near future. Restoration efforts will have to take into account current levels of resistance.
Foxtail (Pinus balfouriana)
Foxtail has the smallest range of any North American five-needle pine species. There are two disjunct populations: one in the Klamath Range in northern California, the other 500 km south in the southern Sierra Nevada. There is little genetic divergence. The climate is warmer and wetter for the northern habitats than for those in the south.
Foxtail pine is second to great basin bristlecone pine in long life, reaching to 3,000 years (Schoettle et al. 2022) The species is experiencing almost no regeneration [Goeking, and Windmuller-Campione 2022].
Foxtail pine is highly susceptible to WPBR; it has greater resistance to MPB (Schoettle et al. 2022; Tomback et al. 2022; Sniezko et al. 2022) WPBR was first reported on the Klamath population in 1967 (Schoettle et al. 2022) The overall health in of the Sierra Nevada population is much better but WPBR is spreading in foxtail pines in Yosemite and Sequoia/Kings Canyon National parks (Nesmith et al. 2022). Before arrival of WPBR, the species had persisted for thousands of years under harsh conditions and provided vital ecosystem services (Hutton, 2015).
Rocky Mountain bristlecone pine (Pinus aristata)
Rocky Mountain bristlecone pine was recognized as a separate species from the Great Basin bristlecone pine in 1970. There is no overlap in their distributions; the core distribution for Rocky Mountain bristlecone pine is in central Colorado into New Mexico with a disjunct population in northern Arizona. Rocky Mountain bristlecone pine grows at high elevation in extensive forests, small stands, and as isolated trees on rocky outcrops. In some locations, it forms spreading krummholz mats above treeline. Rocky Mountain bristlecone pine lives to 2500 years. (Schoettle et al. 2022) Before arrival of WPBR, Rocky Mountain bristlecone pines had persisted for thousands of years under harsh conditions and provided vital ecosystem services (Hutton, 2015).
WPBR was first reported on Rocky Mountain bristlecone pine in 2004 (Schoettle et al. 2022).
To date, only the populations in the Sangre de Cristo Mountains of southern Colorado are infested. Diseased bristlecones are restricted to areas near infected limber pines and Ribes shrubs – which probably heighten inoculum loads. Rocky Mountain bristlecone pines are attacked in similar proportions to limber pine. While Rocky Mountain bristlecone pines are among the most resistant of the North American species, (Sniezko et al. 2022) its protective chemical compounds but in lower concentration than Great Basin bristlecone pines (Bentz et al. 2022).
The USFS DGRC began testing Rocky Mountain bristlecone pines in 2003. (Sniezko et al. 2022).
The greatest threats at present to Rocky Mountain bristlecone pines are fire and shifts in rain patterns (Schoettle et al. 2022).
Great Basin bristlecone pine (Pinus longaeva)
Great basin bristlecone pines grow on isolated mountain ranges and sky islands in 25 ranges in Nevada and eastern California up to southern Idaho [Baker] Great Basin bristlecone pine habitats are warmer and drier than those of Rocky Mountain bristlecone pine, with only a weak pattern of late-summer precipitation and less late winter snow (Schoettle et al. 2022).
Great Basin bristlecone pine is a famously longest-lived tree species; the oldest trees approach 5000 years (Schoettle et al. 2022) There is very little recruitment of the species [Goeking, and Windmuller-Campione 2022].
Great Basin bristlecone pine apparently has higher concentrations of WPBR defensive compounds than other species. (Bentz et al. 2022) WPBR has not yet been detected in the range of Great Basin bristlecone pine (Schoettle et al. 2022; Baker, 2022; Hupp and Galvin, 2022). Fire presents the greatest risk at present (Schoettle et al. 2022).
Great Basin bristlecone pine appears to be a population sink for bark beetles, including but not limited to the mountain pine beetle. Beetle-caused mortality occurs primarily when Great Basin bristlecone pine grows mixed with or near other pine species that support bark beetle population growth (Benz et al. 2022).
—————–
The other high-elevation white pines occur as small, isolated populations; only some are infested (Schwandt and others, 2010). The foxtail pines in the Klamath Mountains of northern California are infested, but the foxtail pines in the Sierra Nevada are not. The only infestation of Rocky Mountain bristlecone pine is in the Sangre de Cristo Mountains of southern Colorado. Diseased bristlecone pine trees are restricted to areas near infected limber pine and Ribes. Great Basin bristlecone pine, including the ancient trees in the California White Mountains, are not infested.
Although blister rust alone can damage white pine, other diseases, insects, and disturbances also pose serious threats (Schwandt and others, 2010). These threats vary by region and stand age and include mountain pine beetle, dwarf mistletoe, and shoot, cone or foliage insects and pathogens. Bear and deer strip bark from the bole; squirrel and other seed predators prevent regeneration. Other factors reducing white pine populations are wildfire and biotic succession.
North American white pine species share a long evolutionary history with the native mountain pine beetle. Blister rust and climate change, however, could greatly affect the character of beetle outbreaks (Hunt and others, 2010). Although most of the mature white pine trees in a stand were killed during an outbreak, enough trees had escaped in the past that populations of white pine could persist. Climate change, however, might allow for more frequent and severe outbreaks, and blister rust might reduce regeneration so much that white pine populations would not recover. The ecological and genetic interactions of beetles with the blister rust pathogen and hosts are so complex that the consequences of management intervention are difficult to predict. For example, a low-intensity burn intended to facilitate pine regeneration might also stimulate Ribes re-growth and thereby increase blister rust incidence (Zambino, 2010).
Genetic variation among American populations of C. ribicola is relatively small (Richardson and others, 2010). Several recent and interesting discoveries have been made, including—genetic differences between eastern and western populations, capacity for a broad telial host range, hybridization, and similarity among blister rust fungi of white pines from Asia, Europe, and North America. Therefore, even though C. ribicola is already widely distributed across North America, new introductions from Eurasia could have major undesirable genetic consequences.
MANAGEMENT
Until the 1960s, Ribes eradication was the principal method for protecting white pine stands from blister rust (Zeglen and others, 2010). Although eradication was at least partially effective, it was discontinued because of increased costs, lower incidence, and conversion to multispecies forest management. Silvicultural methods, including hazard mapping, are still used; but they are augmented with genetic programs to produce improved planting stock. Traditional tree improvement techniques have identified how white pines react to infection (King and others, 2010). The susceptibility of white pine to infection decreases with age (ontogenic resistance). Some trees survive with disease better than others (partial resistance or tolerance). Actively resistant trees (R-gene or major gene resistance) are immune to all but specifically virulent races. Improved stock is available for western white pine and sugar pine; but few stands are now re-planted so opportunities to increase disease resistance during regeneration are lost (Schwandt and others, 2010). New molecular techniques provide the detailed understanding of the biochemistry of resistance needed for conserving high-elevation white pines (Richardson and others, 2010).
Management and research to maintain threatened white pine communities is justified by their ecological and economic importance (Tomback and Achuff, 2010; Schwandt and others, 2010). Some of the biological challenges to sustaining these communities arise from the diversity, complexity, and potential rapid evolution of blister rust pathogens and hosts (Hunt and others, 2010). Constraints on management intervention include costs, policy conflicts, and uncertainty.
Schwandt and others (2010) identify various silviculture and genetics techniques useful for sustaining viable white pine populations on the landscape. For high-elevation white pines, maintaining genetic diversity involves monitoring and protection of designated populations in the wild. Genetic options include selecting for resistance in North American populations and crossing with related Eurasian stone pines with resistance. Although silviculture could enable natural regeneration on many sites, planting would be required on sites lacking a desirable seed source. Adaptive management incorporating participation by a wide range of stakeholders, experimentation, and learning could mitigate the risks of action or inaction and help promote resilient ecosystems.
Several priority conservation actions identified by Hunt and others (2010) are:
- Pathogen biology
- Improve our understanding of Cronartium worldwide, especially genetic origins, migrations, and those aspects of life history and reproductive biology important for assessing its potential for spread and intensification.
- Management by genetic and silvicultural techniques
o Obtain and incorporate into breeding programs the information needed for genetic resource management of white pines, especially high-elevation species
o Continue work on improving pine regeneration, protecting seedlings and poles, and developing better models to assess site hazard and treatment efficacy.
- Knowledge base for deploying these techniques
o Research the ecology, genetics and evolutionary biology of Cronartium ribicola fungi, Ribes and other telial hosts
o Assess and mitigate risk from expanded Ribes cultivation
o Research the genetic and proteomic factors controlling disease expression
- Support management through implementation of monitoring results
o Gain public support for coordinated efforts
o Assess white pine ecosystem conditions, trends and threats, set priorities at regional and local levels; apply mitigation
o Continue providing expertise in forest pathology and other specialized fields.
For further information on this pathogen, please visit:
- US Forest Service, Threat Profile for White Pine Blister Rust (WPBR)
- American Phytopathological Society, WPBR information page
- Forest Pathology, WPBR information
- National Invasive Species Information Center WPBR information
- Whitebark Pine Ecosystem Foundation
REFERENCES
Baker, G.M. 2022. Managing High-Elevation Pines at Great Basin National Park. In Murray, M.P., C.M. Smith, S.T. McKinney, P.L. Achuff. Research and management of five-needle pines in western North America: Proceedings of the second High-Five Conference. 5-7 October 2021. The Press at Cal Poly Humboldt.
Barrett, T.M. and G.C. Robertson, Editors. 2021. Disturbance and Sustainability in Forests of the Western United States. USDA Forest Service Pacific Northwest Research Station. General Technical Report PNW-GTR-992. March 2021
Bentz, B.J., C.I. Millar, J.C. Vandygriff, E.M. Hansen. 2022. Great Basin bristlecone pine mortality: Causal factors and management implications. In Murray, M.P., C.M. Smith, S.T. McKinney, P.L. Achuff. 2022. Research and management of five-needle pines in western North America: Proceedings of the second High-Five Conference. 5-7 October 2021. The Press at Cal Poly Humboldt.
Carerro C. et al. Data sharing for conservation: A standardized checklist of US native tree species and threat assessments to prioritize and coordinate action. Plants People Planet. 2022;1–17. wileyonlinelibrary.com/journal/ppp3
Dawe, D.A., V.S. Peters, and M.D. Flannigan. 2020. Post-fire regeneration of endangered Limber pine (Pinus flexilis) at the Northern extent of its range Forest Ecology and Management 457 (2020) 117725
Dudney, J.C. 2022. The Importance of Energy-Water Limitation Threshold in Drought Impact Studies. In Murray, M.P., C.M. Smith, S.T. McKinney, P.L. Achuff. 2022. Research and management of five-needle pines in western North America: Proceedings of the second High-Five Conference. 5-7 October 2021. The Press at Cal Poly Humboldt.
Goeking, S.A. and D.K. Izlar. 2018. Pinus albicaulis Engelm. (Whitebark Pine) in Mixed-Species Stands throughout Its US Range: Broad-Scale Indicators of Extent and Recent Decline. Forests 20189, 131; doi:10.3390/f9030131
Goeking, S.A. and M.A. Windmuller-Campione. 2022. Comparative Species Assessments of Five-Needle Pines Throughout the Western United States. In Murray, M.P., C.M. Smith, S.T. McKinney, P.L. Achuff. 2022. Research and management of five-needle pines in western North America: Proceedings of the second High-Five Conference. 5-7 October 2021. The Press at Cal Poly Humboldt.
Goheen, E.M. and D.J. Goheen. 2014. Status of Sugar and Western White Pines on Federal Forest Lands in SW OR: Inventory Query and Natural Stand Survey Results. USDA Forest Service Pacific Northwest Region. SWOFIDSC-14-01 January 2014 ,
Guiberson, E. and R. Byron. 2022. Whitebark Pine Restoration in BLM Montana’s Core Areas.Assisted Migration of Whitebark Pine to Higher Latitudes and Elevations near its Northern Limits. In Murray, M.P., C.M. Smith, S.T. McKinney, P.L. Achuff. 2022. Research and management of five-needle pines in western North America: Proceedings of the second High-Five Conference. 5-7 October 2021. The Press at Cal Poly Humboldt.
Geils, B.W.; Hummer, K.E.; Hunt, R.S. 2010. White pines, Ribes, and blister rust: a review and synthesis. Forest Pathology (3/4): 147–185. [Online]. doi: 10.1111/j.1439-0329.2010.00654.x Available: https://www.treesearch.fs.fed.us/pubs/36222
Hansen, A.J., A.East, R.E. Keane, M. Lavin, K. Legg, Z. Holden, C. Toney, and F. Alongi. 2022. Is Whitebark Pine Less Sensitive to Climate Change when climate tolerances of juveniles are considered?
Hooke, J. Whitebark Pine Conservation Program at Crater Lake National Park: 2003-2021. In Murray, M.P., C.M. Smith, S.T. McKinney, P.L. Achuff. 2022. Research and management of five-needle pines in western North America: Proceedings of the second High-Five Conference. 5-7 October 2021. The Press at Cal Poly Humboldt.
Hupp, N. D. Stucki, J. Galvin. 2022. Great Basin Bristlecone and Limber Pine in Great Basin National Park: First Look into the Long-Tern white Pine Monitoring Efforts. In Murray, M.P., C.M. Smith, S.T. McKinney, P.L. Achuff. 2022. Research and management of five-needle pines in western North America: Proceedings of the second High-Five Conference. 5-7 October 2021. The Press at Cal Poly Humboldt.
Hutton, K.M. 2015. A dissertation submitted in partial fulfillment of the requirements for the degree of Doctor of Philosophy. University of Washington.
Hunt, R.S.; Geils, B.W.; Hummer, K.E. 2010. White pines, Ribes, and blister rust: integration and action. Forest Pathology 40 (3–4): 402–417. [Online]. doi: 10.1111/j.1439-329.2010.00665.x Available: https://www.treesearch.fs.fed.us/pubs/36221
Jules, E.S., P.J. van Mantgem, B.G. Iberle, J.C.B. Nesmith, R.M. Rochfort. 2022. An Assessment of Whitebark Pine Populations in National Parks of the Pacific States. In Murray, M.P., C.M. Smith, S.T. McKinney, P.L. Achuff. 2022. Research and management of five-needle pines in western North America: Proceedings of the second High-Five Conference. 5-7 October 2021. The Press at Cal Poly Humboldt.
Keane, R.E., L.M. Holsinger, M.F. Mahalovich, and D.F. Tomback. 2012. Restoring Whitebark Pine in the Face of Climate Change. United States Department of Agriculture Forest Service. Rocky Mountain Research Station. General Technical Report. RMRS-GTR-361
King, J.N.; David, A.; Noshad, D.; Smith, J. 2010. A review of genetic approaches to the management of blister rust in white pines. Forest Pathology 40 (3–4): 292–313. [Online]. doi: 10.1111/j.1439-0329.2010.00659.x
Krakowski, J., J. Gould, M. Berkhout, R. Gutsell. 2022. Hold Your Fire: Whitebark and Limber Pine Regeeration is Not Fire-Dependent in the Canadian Rocky Mountains. In Murray, M.P., C.M. Smith, S.T. McKinney, P.L. Achuff. 2022. Research and management of five-needle pines in western North America: Proceedings of the second High-Five Conference. 5-7 October 2021. The Press at Cal Poly Humboldt.
Meyer, M., M. Slaton, S. Gross, R. Butz, C. Clark. 2022. Ecological Integrity of Whitebark Pine on National Forests in California. In Murray, M.P., C.M. Smith, S.T. McKinney, P.L. Achuff. 2022. Research and management of five-needle pines in western North America: Proceedings of the second High-Five Conference. 5-7 October 2021. The Press at Cal Poly Humboldt.
Milano, E.R., A.G. Vandergast, P.van Mantgem, J. Dudney, J. Nesmith, H. Zald. 2022. Population Structure and Genotype-Environment Associations of Whitebark Pine in the Sierra Nevada.
Murphy, T.E. and M.O. Voigt. Whitebark Pine Tree Improvement and Operational Management on the Helena-Lewis and Clark National Forest. In Murray, M.P., C.M. Smith, S.T. McKinney, P.L. Achuff. 2022. Research and management of five-needle pines in western North America: Proceedings of the second High-Five Conference. 5-7 October 2021. The Press at Cal Poly Humboldt.
Nesmith, J., M. Wright, E. Jules, S. McKinney. 2022. Whitebark Pine in Yosemite, Sequoia, and Kings Canyon National Parks: Assessment of Stand Structure and Condition. In Murray, M.P., C.M. Smith, S.T. McKinney, P.L. Achuff. 2022. Research and management of five-needle pines in western North America: Proceedings of the second High-Five Conference. 5-7 October 2021. The Press at Cal Poly Humboldt.
Novoa, S. Espinosa, B. Fallon, J. Boetsch. 2022. Climatic Factors Affecting White Pine Blister Rust Incidence on Whitebark Pine in Washington National Parks. In Murray, M.P., C.M. Smith, S.T. McKinney, P.L. Achuff. 2022. Research and management of five-needle pines in western North America: Proceedings of the second High-Five Conference. 5-7 October 2021. The Press at Cal Poly Humboldt.
Ostry, M.E.; Laflamme, G.; Katovich, S.A. 2010. Silvicultural approaches for management of eastern white pine to minimize impacts of damaging agents. Forest Pathology 40 (3–4): I332–346. [Online]. doi: 10.1111/j.1439- 0329.2010.00661.x Available: https://www.treesearch.fs.fed.us/pubs/36134
Potter, K.M., Escanferla, M.E., Jetton, R.M., Man, G., Crane, B.S., Prioritizing the conservation needs of US tree spp: Evaluating vulnerability to forest insect and disease threats, Global Ecology and Conservation (2019), doi: https://doi.org/10.1016/
Richardson, B. A.; Ekramoddoullah, A. K. M.; Liu, J.-J.; Kim, M.-S.; Klopfenstein, N.B. 2010. Current and future molecular approaches to investigate the white pine blister rust pathosystem. Forest Pathology 40 (3–4): 314–331. [Online]. doi: 10.1111/j.1439-0329.2010.00660.x Available: https://www.treesearch.fs.fed.us/pubs/36219
Schoettle, A.W., A. Kegley, R.A. Sniezko, K.S. Burns, D. Vogler, P. Peneda Bovin, G. Baker. 2022. Preparing for Invasion: Rust Resistance in Limber, Great Basin Bristlecone, and Rocky Mountain Bristlecone Pines. In Murray, M.P., C.M. Smith, S.T. McKinney, P.L. Achuff. 2022. Research and management of five-needle pines in western North America: Proceedings of the second High-Five Conference. 5-7 October 2021. The Press at Cal Poly Humboldt.
Schoettle, A.W., K.S. Burns, S.T. McKinney, J. Krakowski, K.M. Waring, D.F. Tomback, and M. Davenport. 2022. Integrating forest health conditions and species adaptive capacities to infer future trajectories of the high elevation five-needle white pines. In Murray, M.P., C.M. Smith, S.T. McKinney, P.L. Achuff. 2022. Research and management of five-needle pines in western North America: Proceedings of the second High-Five Conference. 5-7 October 2021. The Press at Cal Poly Humboldt.
Schwandt, J.W.; Lockman, I.B.; Kliejunas, J.T.; Muir, J.A. 2010. Current health issues and management strategies for white pines in the western United States and Canada. Forest Pathology 40 (3/4): 226–250. [Online]. doi: 10.1111/j.1439-0329.2010.00656.x
Shanahan, E. and K. Irvine. 2022. Adaptive Monitoring in Action: Reconsidering Design-based Estimators Reveals Underestimation of Whitebark Pine Disease Prevalence in the Greater Yellowstone Ecosystem. In Murray, M.P., C.M. Smith, S.T. McKinney, P.L. Achuff. 2022. Research and management of five-needle pines in western North America: Proceedings of the second High-Five Conference. 5-7 October 2021. The Press at Cal Poly Humboldt.
Shaw, C.G.; Geils, B.W., guest eds. 2010. Special Issue: White Pines, Ribes and Blister Rust. In: Woodward, S., editor-in-Chief Berlin, Germany: Blackwell Verlag GmbH. [Online]. doi: 10.1111/efp.2010.40.issue-3-4
Sniezko, R.A. pers. comm. September 2022.
Sniezko, R.A. and J-J. Liu. 2022. Genetic resistance to 5-needle blister rust, restoration options, and potential use of biotechnology. Forest Ecology and Management, 520 (15).
Stucki, D. 2022. Challenges to limber pine at Craters of the Moon National Monument and Preserve. Presentation to the Research and Management of High-Elevation Five-Needle Pines in Western North America Second High-Five Conference, October 5 – 7 2021.
Tomback, D.F. 2022. Why a special issue focused on the high elevation five-needles? In Murray, M.P., C.M. Smith, S.T. McKinney, P.L. Achuff. 2022. Research and management of five-needle pines in western North America: Proceedings of the second High-Five Conference. 5-7 October 2021. The Press at Cal Poly Humboldt.
Tomback, D.F.; Achuff, P. 2010. Blister rust and western forest biodiversity: ecology, values and outlook for white pines. Forest Pathology 40 (3/4): 186–225. [Online]. doi: 10.1111/j.1439-0329.2010.00655.x
Tomback, D.F., R.E. Keane, A.W. Schoettle, R.A. Sniezko, M.B. Jenkins, C.R. Nelson, A.D. Bower, C.R. DeMastus, E. Guiberson, J. Krakowski, M.P. Murray, E. R. Pansing, and J. Shamhart. 2022. Tamm review: Current and recommended management practices for the restoration of WB pine (Pinus albicaulis Engelm.), an imperiled high-elevation Western North American forest tree. In Murray, M.P., C.M. Smith, S.T. McKinney, P.L. Achuff. 2022. Research and management of five-needle pines in western North America: Proceedings of the second High-Five Conference. 5-7 October 2021. The Press at Cal Poly Humboldt.
U.S. Fish and Wildlife Service. 2020. Federal Register Vol. 85, No. 232 Wednesday, December 2, 2020
Proposed Rules [Docket No. FWS–R6–ES–2019–0054; FF09E21000 FXES11110900000 212] Endangered and Threatened Wildlife and Plants; Threatened Species Status for Pinus albicaulis (Whitebark Pine) With Section 4(d) Rule
Wendel, G.W. and H.C. Smith https://www.srs.fs.usda.gov/pubs/misc/ag_654/volume_1/pinus/strobus.htm accessed 22-9/16
Zambino, P.J. 2010. Biology and pathology of Ribes and their implications for management of white pine blister rust. Forest Pathology 40 (3/4): 264–291. [Online]. doi: 10.1111/j.1439-0329.2010.00658.x Available: https://www.treesearch.fs.fed.us/pubs/36992
Zeglen, S.; Pronos, J.; Merler, H. 2010. Silvicultural management of white pines in western North America. Forest Pathology 40 (3–4): 347–368. [Online]. doi: 10.1111/j.1439-0329.2010.00662.x